Metallic 3D printing: achieving high-quality results with customized scanning strategies
For 15 years now, researchers at the Dresden branch of the Fraunhofer IWU have been working on the additive technology LPBF (Laser Powder Bed Fusion), in which a laser beam melts metallic powder layer by layer locally at the points where the component grows. In this process, the entire physical and digital process chain must be mastered, to create a functioning component from powder. Adapted scanning strategies that define the sequence, length, alignment, and spacing of the laser paths are a promising approach for LPBF. They help to produce components that are more delicate, homogeneous, dimensionally accurate, and with improved surfaces.
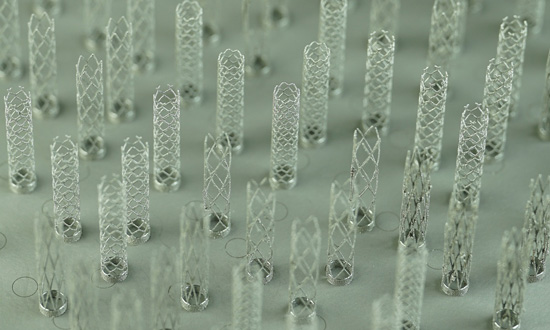
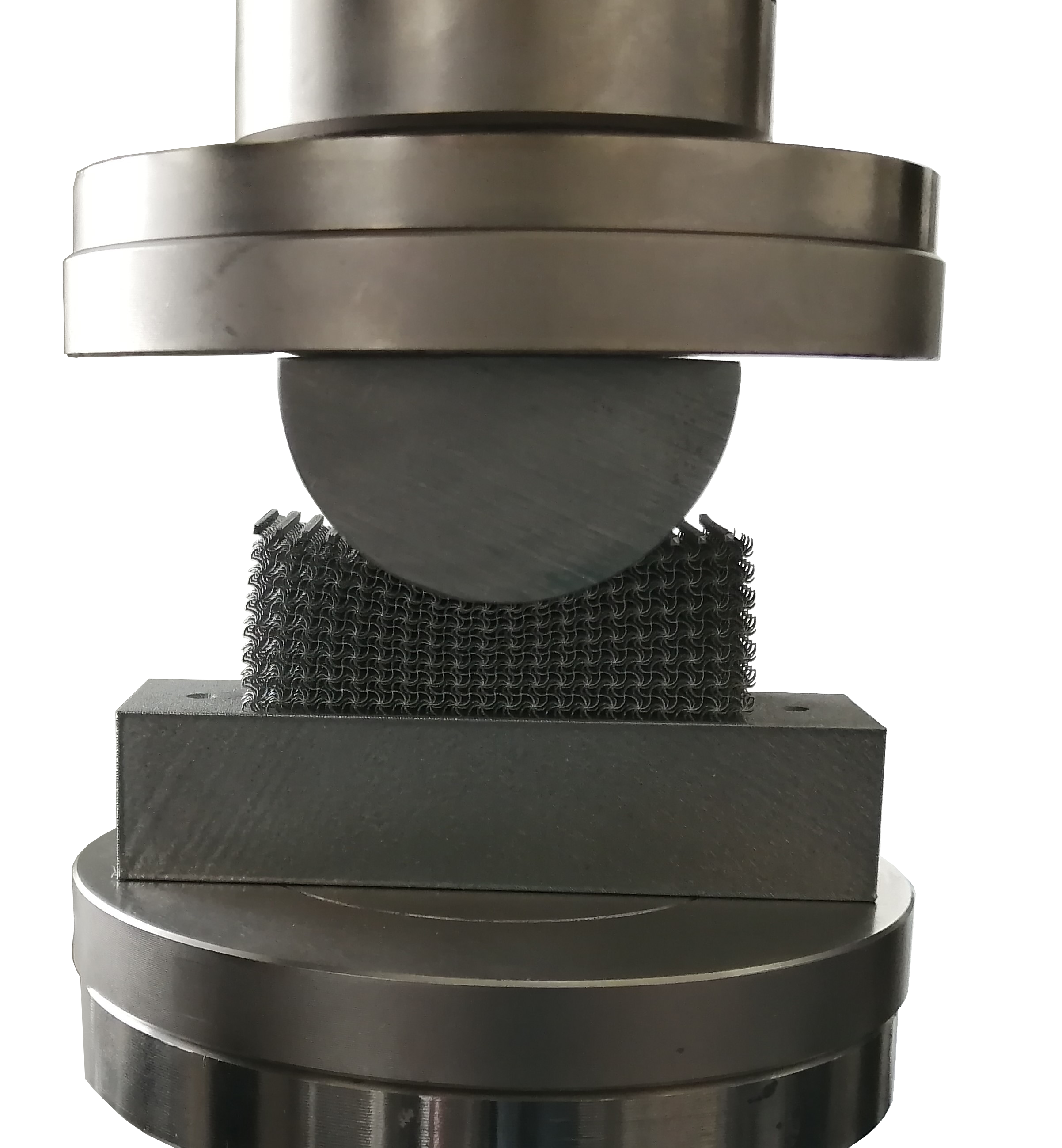
Research and development are carried out in close and long-standing cooperation with the Chair of Virtual Product Development (VPE) at the Technical University of Dresden. A joint software solution now makes it possible to change individual scan paths in a targeted manner and to assign separate parameter sets (laser power, laser speed, etc.) to the scan paths within a component.
Quasi-point scanning strategy for filigree structures
For filigree lattice-like structures, it is necessary to print struts that describe a circle or an ellipse in cross-section, depending on their orientation. Instead of a contour-hatch scan strategy, in which the laser first scans the outer contour and then fills the surface, a so-called quasi-point scanning strategy is used in this application. In this case, only short, partially crossed scan paths are scanned with the laser. This produces much more precise results, especially with delicate components: The energy input is homogeneous in every layer and section, with hardly any build-up. In addition, productivity increases considerably as the laser requires much shorter distances. This procedure can be used, for example, to produce implants with delicate lattice structures such as stents, which keep constricted blood vessels open, particularly in the heart or brain. For the gentlest possible insertion, it makes sense to use superelastic shape memory alloys (SMA), ideally nickel-titanium (NiTi).
Most scanning strategies described are designed for particularly delicate components without support structures and in high surface quality. Future fields of application may lie in medical technology (stents, implants) or clamping technology for sensitive workpieces to avoid point loads.
In addition to applications in medical technology, the additive processing of NiTi for lattice structures has further potential. An internal Fraunhofer research project has developed a clamping and holding element for brittle-fracturing materials such as ceramics. It exhibits locally superelastic behavior and distributes the point load over an enlarged contact surface to prevent critical stresses.
Geometry-adapted scanning strategies for open-pored, cellular structures
Fine, cellular structures required for the production of bone implants can be optimized using a geometry-based scanning strategy during the generation of production data, as shown by the example of a demonstrator of a shoulder short-shaft implant produced at the Fraunhofer IWU. Different areas with specific topographical properties (bridges, cellular, and fully solid areas) are defined using automated geometry recognition. Different scanning strategies and laser parameters are assigned to these sub-areas. Compared to the usual production process (one scanning strategy and one set of laser parameters per component), dimensional accuracy and warpage can be significantly improved. For high dimensional accuracy of cellular structures, it is essential to consider all geometry and process parameters holistically.
Voronoi-based scanning strategies for improved manufacturability of overhang surfaces
The LPBF process allows a high degree of freedom in the design of geometries. The reliable production of high-quality overhang areas (no defects, dimensional accuracy, surface roughness) is particularly challenging. Overhang areas are created when higher levels protrude over lower levels during layer build-up. Support structures – which the component does not require for its function – are often unavoidable.
Together with the Technical University of Dresden (Chair of VPE), Fraunhofer IWU is developing an approach to ensure consistent manufacturing quality with minimal distortion, even in overhang areas, while still operating without modifications to the production equipment and using fewer support structures. The key to success is a combination of automated analysis of the geometry to be produced, thermal simulation of the production process, and a sophisticated Voronoi-based scanning strategy. A closer look at the geometries to be produced reveals areas that require an adjustment of the production parameters: for example, the pattern of the scan paths, partial exposure area sizes, the alignment of scan vectors, or distances between the scan paths. The partial exposure areas are generated using Voronoi diagrams, Voronoi being a mathematical method that divides the scan plane into cells of different sizes based on seed points, i.e. starting points for the growth of the partial exposure areas.
The major advantage here is that the size of the cells can be continuously changed via the distance between the seed points and automatically adapts to the geometry of the component cross-section. To avoid manufacturing errors, the laser parameters should also be adjusted to the geometry-dependent temperature curve during scanning or exposure, with a rough thermal simulation based on a newly developed approach as the basis. Although this leads to slightly less accurate simulation results, it is much faster.
Outlook
Voronoi-based and geometry-adapted scanning strategies offer a universal approach to complex geometry fabrication. The primary objective is to consistently deliver dimensional accuracy, reproducibility, and superior quality across every section within the component. To optimally adapt to diverse geometric conditions, the team at Fraunhofer IWU aspires to achieve a fully automated, self-regulating workflow.
A significant challenge is ensuring optimal heat dissipation, particularly for delicate features such as bridges and thin walls. These require distinct processing techniques compared to solid components. One of the key research directions of the team is the application of these technologies to the demanding requirements of the aerospace industry.
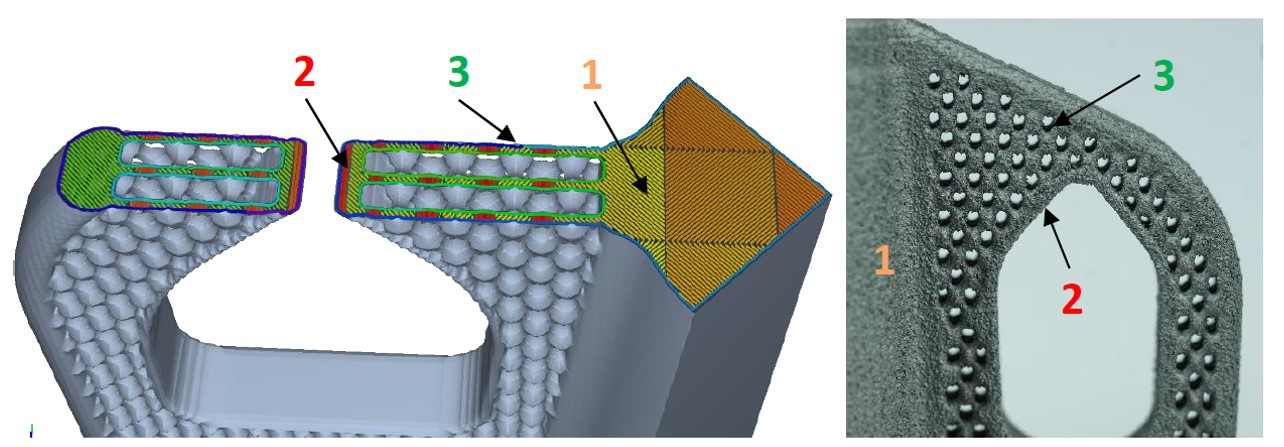
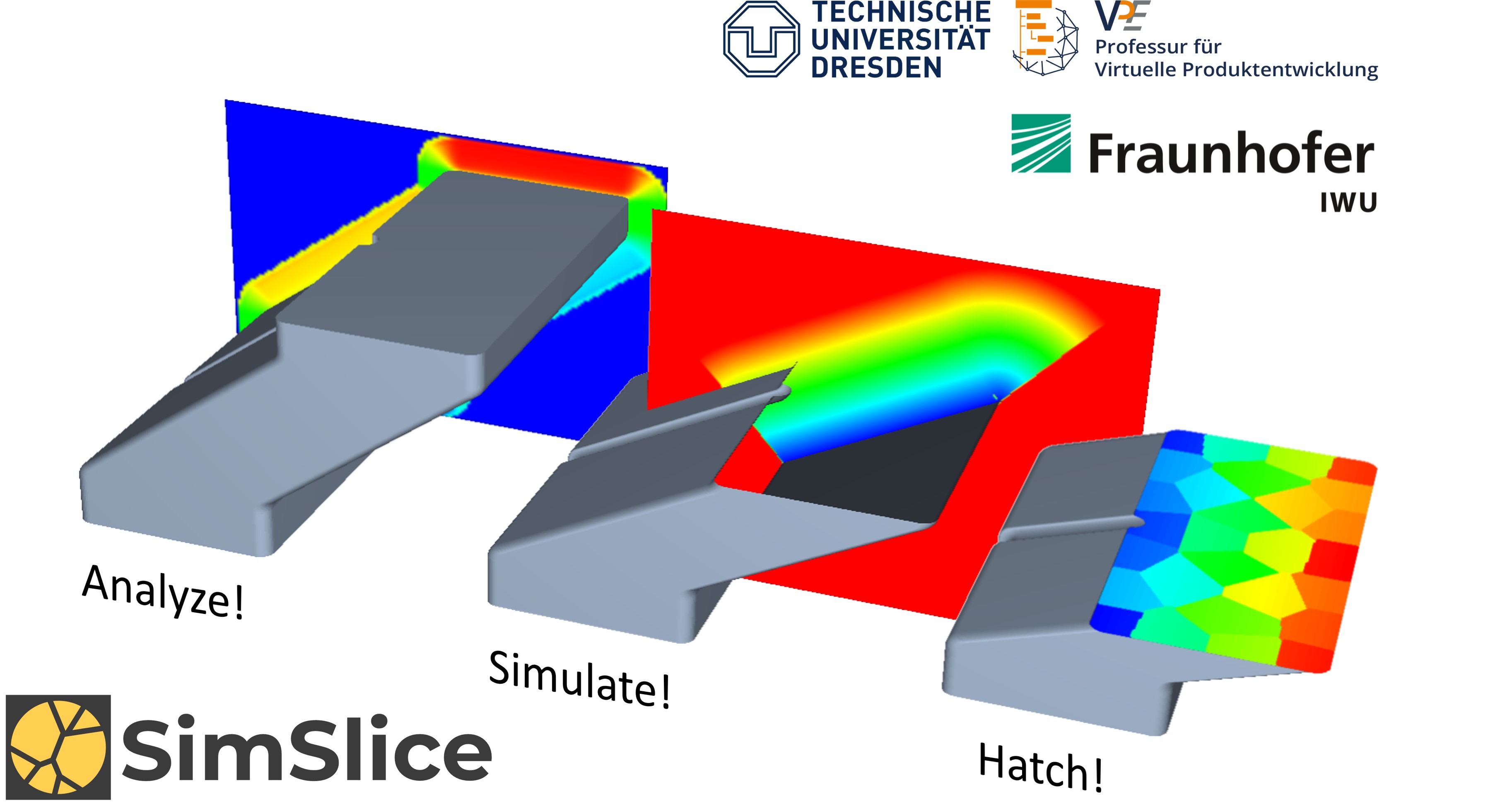